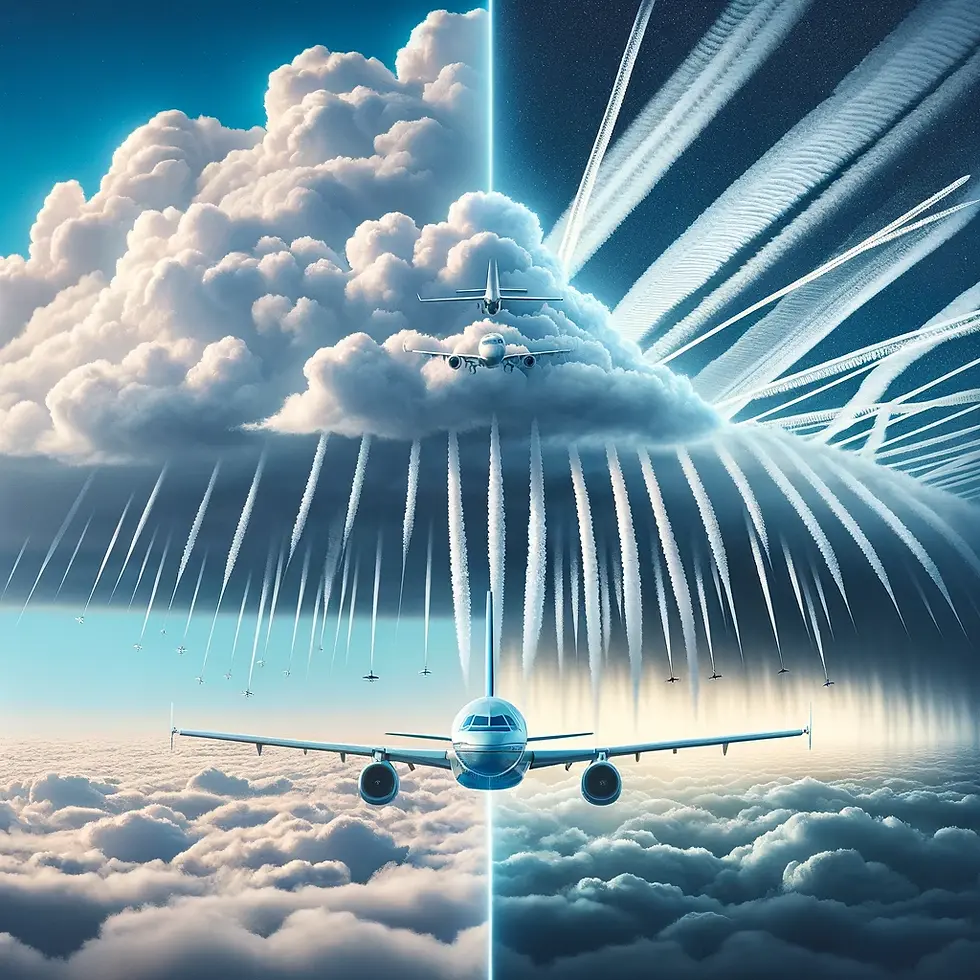
In recent years, the conversation around climate intervention strategies, particularly atmospheric geoengineering, has grown more prevalent. However, without a clear understanding of the long-term outcomes, such extreme measures should be approached with caution. Atmospheric geoengineering, including practices such as cloud seeding, promises to manipulate weather patterns and alleviate environmental challenges. However, the rush to implement these technologies overlooks the potential for long-term consequences that could ultimately exacerbate the problems it aims to solve. Additionally, public discourse is often clouded by the term "chemtrails," a word labeled as "conspiracy theory," which is frequently used to discredit and obscure legitimate discussions about clandestine agendas hidden from the public.
This article seeks to elucidate the differences between cloud seeding and the controversial concept of chemtrails, as well as to discuss the potential unintended effects of geoengineering efforts. Furthermore, it presents the argument that geoengineering should not be viewed as a definitive solution to climate change. Given that climate change has natural components, there is a risk that these interventions might inadvertently trigger the very catastrophes they are intended to prevent.
Climate Change is a Natural Process
Historical and geological evidence shows that the Earth's climate has fluctuated long before human influence. Here are some of the key arguments:
1. Historical Climate Variability: Proponents point to paleoclimatic data showing that the Earth's climate has undergone significant changes over millions of years, including ice ages and warm periods, without any human activity involved.
2. Solar Variability: Changes in solar energy output have been suggested as a natural factor influencing the Earth's climate. Variations in solar radiation, which occur due to sunspot cycles and other solar phenomena, are thought to impact global temperatures.
3. Volcanic Activity: Volcanic eruptions can have a significant impact on climate by emitting large amounts of volcanic ash and sulfur dioxide into the atmosphere, leading to cooling. The argument here is that such natural events can cause climate changes similar to those currently being observed.
4. Earth's Orbital Changes: The Milankovitch cycles, which describe changes in Earth's orbit and tilt, affect the amount and distribution of solar energy received by the Earth and are known to drive long-term climate changes, such as the ice age cycles.
5. Ocean Currents: Changes in ocean currents can significantly alter climate patterns. These currents are influenced by various natural factors and can change over time, affecting regional and global climates.
While these arguments are scientifically valid in explaining that natural factors have historically influenced the Earth's climate, the current consensus among climate change proponents is that the rapid warming observed since the industrial era is predominantly due to increased concentrations of greenhouse gases in the atmosphere, resulting from human activities such as burning fossil fuels and deforestation. However, many could argue that planting trees and burning less fossil fuels is much safer than altering the atmosphere with unknown consequences.
There is substantial scientific evidence from paleoclimatology, the study of past climates, which supports the assertion that there have been periods in Earth's history that were as warm as or warmer than today. Here are some key lines of evidence:
1. Ice Core Data: Ice cores drilled from ice sheets in Greenland, Antarctica, and mountain glaciers provide detailed and direct records of past climate conditions. These cores contain trapped air bubbles that preserve greenhouse gases like CO2 and methane. Analysis of these gases, alongside isotopic data from the ice, shows that there have been periods, such as during the Eocene epoch (about 56 to 34 million years ago) when temperatures were significantly higher than today.
2. Sedimentary Records: Sediments deposited over millions of years on ocean floors and lake beds also contain materials that help reconstruct past climates. For example, the ratios of certain oxygen isotopes in marine fossils (like foraminifera) reflect ancient ocean temperatures and ice volumes. These records have shown warm periods such as the Mesozoic era, particularly during the Cretaceous period (about 145 to 66 million years ago), which was warmer than today.
3. Geological Evidence: Various geological formations and fossils indicate past climate conditions. For instance, the presence of coal deposits in Antarctica suggests that the continent once hosted dense forests, implying much warmer global conditions.
4. Paleobotanical Data: The distribution of fossil plants and pollen types over geological timescales also provides insights into past climates. Certain plant species that are indicative of warm climates have been found in regions now considered temperate or even polar, suggesting these regions were once much warmer.
5. Paleogeographic Reconstructions: The movement of tectonic plates has caused the positions of continents and ocean layouts to shift over millions of years. These changes affect ocean currents and climate. For example, during the Permian period (about 299 to 251 million years ago), the supercontinent Pangea had a climate that was extremely different from today’s climate, with interior regions experiencing extreme temperatures.
While these and other methods have demonstrated that there were periods in Earth's history warmer than today, it's crucial to understand the context and causes behind those temperatures. These past warm periods were often associated with higher levels of greenhouse gases from natural sources and occurred over geological timescales.
Geoengineering is a Risky Solution to Counteract a Natural Process Like Climate Change
Geoengineering, specifically the intentional large-scale manipulation of the Earth's climate system, is often proposed as a method to counteract the effects of climate change. However, it is highly contentious, with numerous potential risks and unknown long-term consequences. Here are some arguments against pursuing geoengineering as a primary solution to climate change:
1. Unknown Ecological Impacts: Geoengineering techniques, such as stratospheric aerosol injection, which aims to cool the planet by reflecting sunlight away from Earth, could have unforeseen ecological consequences. For instance, altering the amount of solar radiation that reaches the Earth could disrupt weather patterns, affecting agriculture and water resources. These changes could have a disproportionate impact on different regions, potentially causing droughts or extreme weather in areas dependent on consistent climate conditions.
2. Biodiversity Risks: By altering the climate quickly through geoengineering, ecosystems may not have enough time to adapt, leading to a loss of biodiversity. Many species already stressed by existing climate changes could find themselves even further threatened by rapid shifts in climate conditions, potentially leading to extinction events.
3. Moral Hazard: Relying on geoengineering could lead to a "moral hazard," where the perception of a quick technological fix might reduce the urgency of reducing greenhouse gas emissions. This could delay essential mitigation efforts such as energy efficiency, renewable energy development, and conservation practices, which are more sustainable and less risky in the long term.
4. Reversibility and Dependence: Some geoengineering approaches may not be easily reversible once started. For example, if aerosol injections were used and suddenly halted, there could be rapid and potentially catastrophic warming known as "termination shock." This dependency on continued intervention could bind humanity to an unsustainable path of constant geoengineering.
5. Ethical Concerns: There are significant ethical issues regarding the impacts of geoengineering on future generations. Implementing such technologies without fully understanding their effects risks leaving a legacy of environmental and social challenges that could affect countless generations.
Given these concerns, many argue that the focus should remain on more natural and less risky solutions to climate change, such as enhancing carbon sinks through reforestation and drastically reducing fossil fuel use. These methods have clearer benefits and fewer potential negative side effects, aligning more closely with the precautionary principle that advocates for the avoidance of actions that can cause potentially serious or irreversible damage.
Current Geoengineering Research and Projects
Several types of geoengineering research and projects are being explored. The two main categories are Solar Radiation Management (SRM) and Carbon Dioxide Removal (CDR). Here's a brief overview of some of the practices under these categories:
Solar Radiation Management (SRM)
1. Stratospheric Aerosol Injection (SAI): This involves injecting particles, such as sulphate aerosols, into the stratosphere to reflect sunlight and cool the Earth.
2. Marine Cloud Brightening: This technique aims to increase the reflectivity of clouds over the ocean by spraying seawater to encourage more cloud formation, which would reflect more sunlight back into space.
3. Space-based Reflectors: Theoretically, this involves placing large mirrors or other reflective objects in space to reduce the amount of solar energy reaching Earth. It remains in development due to its high cost and technical challenges.
Carbon Dioxide Removal (CDR)
1. Afforestation and Reforestation: Increasing the number of trees and forests on Earth can naturally remove CO₂ from the atmosphere through photosynthesis. This is the most widely used form of geoengineering, as it is a natural process and has additional benefits for biodiversity and soil quality.
2. Bioenergy with Carbon Capture and Storage (BECCS): This process involves growing biomass, burning it for energy, capturing the CO₂ produced during the burning process, and sequestering it underground. While there are operational projects, its scalability is limited by land use, water requirements, and ecological impacts.
3. Direct Air Capture (DAC): This technology captures CO₂ directly from the atmosphere using chemical processes. The captured CO₂ can then be stored underground or used in various industrial processes. Several pilot projects and small-scale facilities are operational around the world.
4. Enhanced Weathering: This involves spreading finely ground silicate minerals on the land surface to accelerate the natural process of weathering, which captures atmospheric CO₂ and converts it into stable minerals.
5. Ocean Fertilization: This involves adding nutrients to the ocean, such as iron or phosphorus, to stimulate the growth of phytoplankton, which absorbs CO₂. Due to concerns about its ecological impacts, including potential disruption to marine ecosystems, it is currently limited to small-scale research trials.
While some of these practices, particularly those involving carbon removal, are used to varying extents, major SRM techniques remain largely at the research or conceptual stage. The deployment of such technologies, especially on a large scale, would require rigorous international governance frameworks to manage risks and ensure equitable impacts globally.
Understanding Cloud Seeding
Cloud seeding is a form of weather modification that enhances precipitation processes and is aimed at increasing rain or snowfall amounts, reducing hail damage, or dispersing fog. The technique involves the aerial dispersion of substances like silver iodide, potassium iodide, or frozen carbon dioxide into the upper parts of clouds. These particles serve as nuclei around which moisture can condense, eventually growing large enough to fall as precipitation.
This method has been in practice since the 1940s and has been an operational application across various countries, including the United States, China, Australia, and European Countries. It is typically used to alleviate drought conditions, enhance water supplies, or decrease wildfire risks by increasing humidity levels within specific regions. It's one of the most commonly used geoengineering techniques.
How Cloud Seeding Works
Cloud seeding involves the introduction of substances into clouds that encourage the formation of ice or water droplets, leading to precipitation. Common substances used for seeding include:
Silver iodide: Resembles the structure of ice, making it effective in encouraging ice crystal formation in cold clouds. Silver iodide is known to be toxic and is regulated under the Clean Water Act as a hazardous substance.
Sodium chloride (table salt) or other salts: Used to seed warm clouds to encourage droplet growth. Commonly known as table salt, this ionic compound also serves as a densifier in the production of artificial rain. When used in cloud seeding, sodium chloride helps to increase the cloud's density with larger water droplets, which promotes precipitation and causes rain to fall onto the Earth's surface. Although effective, the use of sodium chloride is typically more expensive than silver iodide and is primarily employed during severe dry seasons.
Potassium iodide: Also used in various types of cloud seeding efforts to create artificial rain, but it is also has other uses. Potasium iodide function as a photosensitizer with silver nitrate. Potassium iodide is used as a shield against nuclear radiation.
Carbon Dioxide: CO2 is used in cloud seeding in the form of dry ice. Inthe form of ice, CO2 helps weigh down the clouds and press the trapped water to fall down to surface of the earth.
Propane: Propane, C3H8, is a common substance used as fuel is a natural gas. In cloud seeding C3H8 encourages cloud precipitation.
Calcium Carbide: In the process of producing artificial rain, calcium carbide is utilized during the agitation phase. This chemical, a calcium compound with the formula CaC2, often appears colorless but may also have a white-ish hue. It is created by reacting a mixture of lime and coke in an electric arc furnace, yielding calcium carbide. In combination with calcium oxide, calcium carbide serves as a water vapor absorber. It typically includes additives like salt, urea, or ammonium nitrate. Its primary function in artificial rain creation is to initiate precipitation within cloud formations in the sky.
Ammonium Nitrate: Ammonium nitrate, another key chemical used in cloud seeding, is the nitrate salt of the ammonium cation and has the chemical formula NH4NO3. It typically appears as a white crystalline solid and is commonly used as a fertilizer in agriculture. However, ammonium nitrate also plays a crucial role in the cloud seeding process for creating artificial rain. It acts alongside other chemicals to increase the number of nuclei, or water droplets, within clouds, thereby enhancing the clouds' density. This function is vital as these nuclei are the droplets that eventually fall as rain.
Urea Compound: Urea, commonly utilized in agriculture, also plays a significant role in cloud seeding. Known chemically as carbamide, with the formula CH4N2O, urea is a white solid typically used as a fertilizer for plants. In the context of cloud seeding, urea functions as a water vapor absorber. This action helps to condense the water vapor, forming denser clouds that can more effectively precipitate. Additionally, urea stimulates the transformation of crystallized water within the clouds into liquid droplets.
Applications and Objectives
1. Increasing Rainfall: Cloud seeding is frequently used in areas experiencing drought or water scarcity. The aim is to enhance natural precipitation processes, increasing water availability for agriculture, drinking, and other uses.
2. Snow Enhancement: Particularly in mountainous regions, cloud seeding is employed to increase snowfall, which can benefit water supplies and winter sports industries.
3. Hail Suppression: Some cloud seeding efforts aim to reduce the size of hailstones before they reach the ground, helping to prevent damage to crops, vehicles, and property.
4. Fog Dissipation: Airports and transportation authorities sometimes use cloud seeding to clear fog, improving visibility and safety for aircraft and ground transportation.
Cloud Seeding and Its Association with Chemtrails: Are they the Same Thing?
The chemtrails conspiracy theory holds that the visible trails left by aircraft are not simple contrails—condensation trails formed when hot airplane exhaust meets cold, high-altitude air—but rather, are composed of chemicals or biological agents deliberately sprayed by governments or other entities for secretive purposes. This theory has been fueled by persistent denials from governments regarding any such activities, despite what theorists argue is visible evidence to the contrary. Observers point to grid-like formations in the sky, which they believe could not result from routine air traffic patterns and suggest deliberate, large-scale chemical dispersion.
Chemtrails, as a conspiracy theory, could stem from a lack of transparency about atomospheric geoengineering practices like cloud seeding. This is exacerbated by the visual impact of persistent trails, which can spread and contribute to cloud formation under certain atmospheric conditions, thereby appearing as an unnatural cloud cover. This spectacle, coupled with insufficient public communication and official discussions about cloud seeding and other geoengineering practices, has inadvertently provided a fertile ground for conspiracy theories.
To address and reduce speculation, it is crucial for governments and the media to improve transparency regarding geoengineering efforts. Educating the public about the nature of geoengineering and the regulatory oversight of air traffic (domestic vs. geoengineering efforts) could help clarify these widespread speculation of nefarious spraying of chemicals and biological agents.
Effectiveness and Controversy
The effectiveness of cloud seeding is subject to ongoing debate. Variables such as cloud type, atmospheric conditions, and seeding techniques play critical roles in determining its success. Furthermore, environmental and regulatory concerns exist, including the impacts of chemical agents on ecosystems and the potential for conflicts over water rights and cross-border precipitation effects. Despite these issues, cloud seeding is employed in various regions around the world, including parts of the United States, Australia, Europe, China, India, and the Middle East.
The Risks of Manipulating Nature
At the heart of the debate on atmospheric geoengineering is the fundamental uncertainty about the long-term impacts of intervening in complex natural systems. Cloud seeding, for instance, involves injecting substances like silver iodide into clouds to encourage precipitation. While this can increase rainfall in drought-prone areas, the broader ecological implications remain poorly understood. Altering precipitation patterns can disrupt local ecosystems, impact water cycles, and lead to unintended weather extremes in other regions due to the interconnected nature of global weather systems.
The modification of one aspect of the environment invariably affects others in unpredictable ways. For example, increasing rainfall in one area might reduce water availability downstream or alter agricultural patterns due to changes in soil moisture levels. Such interventions could create new environmental stresses, potentially leading to biodiversity loss or new conflicts over water rights.
Ethical and Social Implications
Beyond ecological concerns, the ethical implications of atmospheric geoengineering are profound. Deciding to artificially alter weather patterns raises questions about consent and justice. Who decides when and where to deploy these technologies? Which communities will bear the risks, and who will reap the benefits? Often, the regions most affected by climate are those with the least influence over global environmental policies, potentially deepening existing inequalities.
Moreover, reliance on technological fixes may divert attention and resources from more sustainable solutions to climate change, such as reducing carbon emissions and protecting natural ecosystems. There is a real danger that geoengineering could be seen as a substitute for these more difficult, yet ultimately more sustainable, policy changes.
The "Slippery Slope" of Geoengineering
The concept of a "slippery slope" is particularly relevant to the discussion on atmospheric geoengineering. Once started, such interventions might become increasingly normalized, potentially leading to a scenario where escalating interventions are required to counterbalance previous actions or unintended side effects. This could trap humanity in a cycle of dependency on technology to maintain or control climate conditions, each step removing us further from natural environmental processes.
The Call for Caution
The potential for unforeseen and possibly irreversible impacts makes it imperative to prioritize a deeper understanding of these technologies' long-term effects over their immediate deployment.
As a society, we must weigh the risks of deploying imperfect and potentially dangerous technologies against the benefits of investing in sustainable and natural solutions to environmental challenges. The focus should remain on reducing human impact on the environment through emission reductions, conservation efforts, and sustainable practices rather than relying on uncertain technological fixes that may create new problems in the future.
Comments